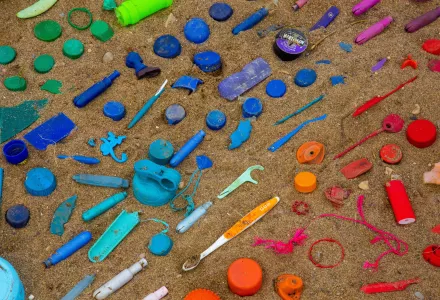
This paper was published in the Journal of Self-Governance and Management Economics in February 2022.
Abstract
The ongoing COVID-19 pandemic is upending our lives and the global economy in ways unimaginable until recently. While the overall impacts are still difficult to quantify, ramifications are sure to be felt for decades to come. Providing secure, reliable, and affordable resources for all without causing devastating environmental consequences is perhaps the greatest challenge of the 21st century. But the pandemic has significantly altered dynamics and changed priorities. How is this impacting the quest for sustainability?
In this paper we analyze these challenges by focusing on the plastic industry. There is no doubt that plastic has molded society in many ways that make our lives easier and safer, but it has also created a global environmental and sustainability crisis. In order to curb our addiction to plastic, the world had been waging a war against virgin plastic, but the pandemic has turned an enemy into a much-needed ally. How can we leverage the advantages of plastic without contributing to the world’s environmental crisis? This dilemma poses a significant challenge, but also opens an opportunity to address sustainability at a systemic level through circularity and the transition to low-carbon alternatives to petroleum-based plastics.
Introduction
“Material invented in the early 20th century, which has enabled modern life as we know it, and whose sustainable production and disposal is one of the major challenges of our century.” Crosswords lovers will undoubtedly recognize this clue for a seven-letter word.
But when we think of plastic, what really does spring to mind? Plastic has become so ubiquitous in our daily lives that we do not give it much thought. Yet it is everywhere: from clothing to chewing gum, from tea bags to whitening toothpaste, from toys to plastic bags or bottles. Up to five trillion single-use plastic bags are consumed every year and one million plastic water bottles are sold each minute globally.1 However, the lack of end-of-life considerations and the high costs of recycling2 have turned plastic into the victim of its own success by creating a global environmental crisis. Plastic lies at the center of society’s debate on sustainability, even more so during the ongoing COVID-19 pandemic, which has turned plastic into a much-needed ally.
Since the inception of the plastic industry, more than 6.3 billion tons of plastic waste have been produced worldwide,3 the vast majority of which ended up in landfills. According to a Pew Charitable Trust study, approximately 11 million metric tons of plastic enter the oceans each year, an amount that will triple by 2040.4 On the current trajectory, there will be more plastic in the oceans by weight than fish by 2050,5 and plastic can stay in the environment for hundreds of years—and even when degraded, it never truly leaves our ecosystems, but remains present as smaller pieces or particles. Once in the ocean, microplastics can commonly be mistaken for plankton and are ingested by marine life, leading to choking, starvation and propagation along the food chain.6 Furthermore, plastic has a significant carbon footprint not only because production is energy intensive, but also because it is made from of fossil fuels. In 2019, the production and incineration of plastic generated about 850 million metric tons of greenhouse gasses (GHG)—equal to the emissions of 189 five-hundred-megawatt coal power plants7 or equivalent to2.6 % of energy related global GHG emissions.8
Two main reasons are at the basis of this global waste crisis:
First, a linear economy approach (see Figure 1), also called a “take-make-use-waste” value chain, in which raw materials are transformed into products that are then used until being thrown away as waste. This model creates value by manufacturing and selling as many products as possible, at the cheapest cost possible, and assumes that the planet’s resources are infinite. As a result, it does not take into consideration any end-of-life issues.
Second, today’s plastic economy is highly fragmented. To begin with, not all plastics are equal, as they have different physical-chemical properties and lifespans. These differences are exacerbated by the lack of global standards and coordination across the value chain, which has allowed the proliferation of different materials, labelling, collection schemes, sorting and reprocessing systems, all of which hinder overall sustainability. Furthermore, innovation activities are also fragmented—the design of new materials is often disconnected from the development and deployment of after-use processes and infrastructure.
A new plastic paradigm is therefore needed. Achieving a long-term sustainable future for plastic will require integration along the entire value chain, from design to reuse, together with the transition to a truly circular economy. A circular economy is characterized by a value chain approach in which a product’s end-of-life is taken into consideration from the moment it is developed, and resources are reused instead of being continuously added (see Figure 2). In terms of innovation, this will also require an increased focus on composition, both in terms of smarter materials design to improve recyclability and the development of bio-based alternatives.
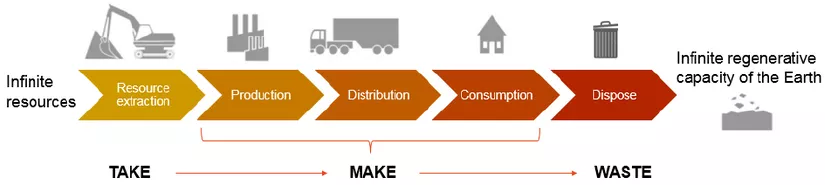
Figure 1. Linear Economy Value Chain Approach (Wautelet 2018)9
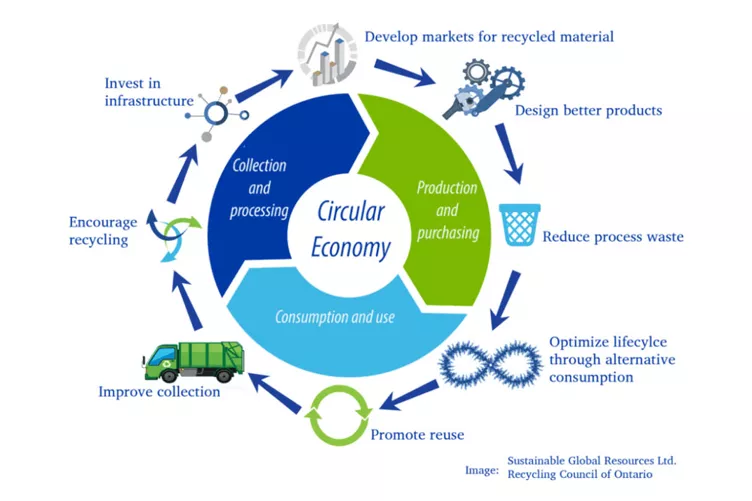
Figure 2. Circular Economy Value Chain Approach (Recycling Council of Ontario 2020)
Before the spread of COVID-19, policy and regulatory actions had begun to address the ramifications of a linear economy approach by mandating reductions in single-use plastic consumption, increasing recycling, and developing bio-alternatives to petroleum-based materials.
However, the COVID-19 pandemic has disrupted all these efforts. Demand for personal protective equipment (PPE), safety screens, and single-use plastic products, such as takeout containers, plastic bags, and packaging has skyrocketed. Regulators have postponed or lifted bans, prohibited consumers from using reusable items,10 and interrupted recycling services to prevent cross-contamination.11 At the same time, energy demand has plummeted in many sectors, shortly followed by commodity prices—making petroleum-based virgin plastic even less expensive.12
These dynamics have given plastic a new lifeline, but if the associated environmental challenges are not properly addressed the world will be facing a new pandemic—a plastic pandemic.
While the exact impacts are difficult to quantify as the COVID-19 pandemic is ongoing, the implications are sure to be felt for decades to come. What is certain is that plastic cannot simply be extricated from our daily lives. The question then becomes: what does a sustainable future for plastic look like?
Our study explores the opportunities and challenges associated with the transition of the plastic value chain to a circular economy and provides recommendations on how to accelerate this process.
The remainder of this paper is structured as follows: Section 2 describes how the world was approaching the sustainability and environmental challenges of plastic prior to the COVID-19 pandemic. Section 3 analyzes how these efforts were upended by the pandemic. Sections 4 and 5 give an overview on what plastic is and the challenges of recycling. Section 6 provides an outlook on the sustainable future of plastic, and Section 7 presents overall conclusions and recommendations.
Plastic before COVID-19
Prior to the COVID-19 outbreak, the challenges associated with plastics—poor waste management, end-of-life concerns, and associated carbon footprint—were gaining traction as urgent issues to be addressed for a more sustainable future. The world had finally started to move towards value chains distinctive of a circular economy—and while far from comprehensive, tangible measures had begun to mitigate the environmental impacts of the old linear economy approach.
Governments all over the world were introducing legislations and initiatives to improve plastic waste management, to ban single-use items such as shopping bags and straws, and to find alternatives to petroleum-based plastic.1314 In the private sector, companies such as PepsiCo and Coca-Cola, for example were setting voluntary targets to increase the use of recycled plastics in their operations.15
The United States (US), European Union (EU), and the United Nations (UN) all have policies to support circularity in the name of sustainability. In the US, where circularity is referred to as “sustainable materials management”, the Environmental Protection Agency (EPA) has implemented programs to support advanced waste management strategies and materials production with more efficient and sustainable life cycles.16 In 2015, the EU enacted its Circular Economy Action Plan,17 which includes measures to boost global competitiveness, foster sustainable economic growth, and generate new jobs. Furthermore, the 2018 revision set clear targets for waste reduction and established an ambitious long-term path for waste management and recycling—including recycling 55% of plastic packaging by 2030.1819
In 2015, the UN adopted its 2030 Agenda for Sustainable Development,20 a blueprint to achieve a better and more sustainable future for all, at the heart of which are seventeen Sustainable Development Goals (SDGs). The approach for achieving many of these goals either directly or indirectly draws on the philosophy of circularity, but even before the COVID-19 pandemic much still needed to be done to achieve them. A 2019 progress report stated that “according to current trends, global resource use will continue to grow, reaching over 18 tons per capita by 2060, with correspondingly increased levels of greenhouse gas emissions (43 per cent relative to 2015), industrial water withdrawals (up to 100 per cent relative to 2010) and agricultural land area (up to 20 per cent relative to 2015), with a reduction in forests by over 10 per cent.”21
Stakeholders and Non-Governmental Organizations (NGOs) around the world were also increasingly pressing the private sector into action. In 2016, the Ellen MacArthur Foundation launched the New Plastics Economy Initiative, aimed at accelerating the transition to value chains typical of a circular economy, to address the environmental impacts of plastic production and use. In responseglobal players, such as Danone, Walmart and Amcor, pledged to use their manufacturing and purchasing power to transition away from virgin, single-use plastics.22 Amcor, a multinational packaging company in the food, medical, home and personal care sectors, for example committed to designing all of its products so that they are either recyclable or reusable, and increasing the use of post-consumer recycled materials to 10%, by 2025. According to Amcor’s 2019 Sustainability Report, the company was able to eliminate almost 17,000 tons of plastic waste by redesigning its products, while at the same time decreasing water use by 8.7% and greenhouse gas emissions by 8.6%.23
It is important to notice that this increased focus on plastic composition was not only limited to smarter designs to improve recyclability, but also to the development of bio-based alternatives to traditional plastic, as discussed in more detail later in this paper.
Although progress was not uniform or particularly fast-paced, the environmental crisis associated with plastic production, use and disposal had finally captured the attention of the public, governments, and businesses around the world. The search for sustainable solutions which could be scaled up had begun, together with the recognition that addressing the symptoms of this crisis through simple clean-ups was not enough, but that a systemic shift tackling the root causes of this environmental and sustainability crisis was required.
However, the COVID-19 pandemic has significantly disrupted not only our lives but also how the world sees plastic. Personal protective equipment (PPE) and other plastic products have become key in preventing the spread of the virus, thus increasing demand and making the need to address the sustainable future of plastic even more pressing.
Plastic and the Pandemic
As COVID-19 spread across the globe, nations found themselves underprepared and ill-equipped to weather the pandemic.Healthcare workers rely on PPEs such as face masks, gloves, gowns, goggles, and face shields to protect themselves and their patients from being infected and infecting others. PPEs shortages caused by skyrocketing demand, panic buying, hoarding and misuse left frontline workers dangerously under protected to fight the pandemic—putting lives at risk.
At the height of the first COVID-19 wave, one single hospital in New York city had to increase its orders for face masks from 20,000 to 300,000 per week.24 At a global level, the World Health Organization estimates that 89 million surgical masks, 76 million examination gloves, and 1.6 million goggles will be required to respond to the COVID19 crisis each month; and that to meet this demand, manufacturing capacity will need to increase by 40%.25
But why is this relevant to the plastic industry? Because PPEs are mainly made of plastic. Surgical and N95 masks are made of polypropylene or polystyrene,26 medical gloves of latex or polyvinyl chloride,27 goggles of polycarbonate,28 protective gowns of polyester or polyester-cotton blends.29 And the list continues—hand sanitizers and disinfecting products contain plastic and/or are sold inside plastic packaging.
As businesses cautiously try to reopen, plastic screens to maintain social distance guidelines and single-use plastic containers for food and beverages have become a must. In the United States, the Centers for Disease Control and Prevention (CDC) encourages the service industry to use disposable menus, plates and utensils, together with single-serving condiment containers.30 Some states and municipalities have gone even further, either by postponing the enactment of bans on single-use plastic products or temporarily lifting those that were already in place and instead, banning businesses from allowing customers to bring their own reusable bags or mugs.31Although these measures are meant to protect our health and safety, they are also bolstering an already dangerous reliance on single-use plastic.
At the same time, due to the lockdowns—coupled with decreased demand—fossil fuels prices have plummeted, making virgin plastic a much more profitable alternative to using recycled plastic.32 And while the former has always been the less expensive option, it is now even more enticing to producers, hindering the transition to more sustainable business models.
Furthermore, even before the pandemic low recycling levels made it difficult for companies to source the materials needed for sustainability initiatives. According to the Consumer Brand Association, in 2019 there was only enough recycled plastic in the US to meet 6% of demand.33 Companies are thus faced with the challenging choice of either maintaining their sustainability plans or adding to the industry’s environmental challenge by choosing the more profitable option. Citing the pandemic as the reason, companies like Danone and Coca-Cola have already missed their self-imposed sustainability goals for this year.34
The pandemic has also deeply affected waste management and recycling schemes throughout the world. While PPEs from healthcare services are disposed of in sealable bags (more plastic) before being incinerated as medical waste,35 individuals are advised to discard PPEs with domestic waste,36 making it potentially biohazardous for trash collectors. Unfortunately, this is not enough to guarantee that the regular plastic waste being recycled has also not been contaminated making recycling impossible and increasing the burden on already strained waste management systems.
During the complete shutdowns endured in many countries, services that were already poorly managed, such as recycling and can collection, were completely halted. In the US alone, at least 100 municipalities suspended curbside recycling programs as COVID-19 cases surged.37 Even after the shutdowns, most recycling operations have struggled to get back to full capacity due to the challenge of protecting workers from potentially harmful waste. During the first week of August 2020, recycling services in Houston, Philadelphia, and Nashville, among others, reported disruptions due to increasing volumes and reduced staff.38 As a result an increasing number of items which under normal circumstances could be recycled are instead sent to landfills, adding to the sustainability challenge.
The pandemic has turned plastic into a key ally to fight COVID-19 but has also created a perfect storm for petroleum-based plastic pollution: demand is high, costs are low, and unfortunately, municipal waste services are not equipped to process potentially contaminated plastic waste.
What is Plastic?
We usually talk about “plastic” as though it is a single material, but as mentioned, there are many different kinds. The word plastic derives from the Latin “plasticus”, meaning capable of being molded into various forms and refers to a wide range of synthetic and natural materials with many versatile properties and potential applications.
Plastics are inexpensive, durable, and lightweight. They can be soft and pliable or hard and strong, they can withstand extreme cold or heat, and they can be corrosion or chemical resistant. Furthermore, if the physical properties do not meet the desired requirements, they can be enhanced with additives such as pigments, plasticizers, flame retardants, antioxidants, light and heat stabilizers, lubricants, antistatic agents, etc.39
Interest in plastic arose in the 1800s to replace scarce materials such as ivory and horn. The first synthetic plastics were derived from cellulose, a substance found in plants.40 Today, plastics are one of the staple products of the chemical industry and are mainly produced from fossil fuels, with only less than 1% deriving from biobased materials such sugar, starch, or plant-based oils.41 Global production is constantly increasing and reached almost 360 million tons in 2018. China was by far the world’s largest producer, with a market share of 30% (108 million tons), followed by North America at 18% (65 million tons) and Europe at 17% (62 million tons).42 Demand by sector varies from country to country, but packaging and construction usually dominate. Shares for the EU’s market were as follows: packaging 39.9%, building and construction 19.8%, automotive 9.9%, electrical and electronics 6.2%, household, leisure and sports 4.1%, agriculture 3.4%, others (appliances, mechanical engineering, furniture, medical, etc.) 16.7%.43
Plastics are made of one or more polymers44 and include well-known names such as PE (polyethylene) used in plastic bags, trays and containers, food packaging, etc.; PP (polypropylene) used in food packaging, snack wrappers, hinged caps, microwave containers, pipes, automotive parts, bank notes, etc.; PVC (polyvinyl chloride) used for window frames, floor and wall covering, pipes, cable insulation, garden hoses, inflatable pools, etc.; PET (polyethylene terephthalate) used for bottles for water, soft drinks, juices, cleaners, etc.; PS (polystyrene) used for Styrofoam cups, building insulation, electrical and electronic equipment, inner liner for fridges, eyeglasses frames, etc.; and Teflon (polytetrafluoroethylene) used for nonstick surfaces.
Synthetic plastics can be broadly divided into two categories: thermoplastics and thermosets.45Thermoplastics remain plastic after molding and can therefore be reshaped and recycled repeatedly. Thermosets, on the other hand, undergo a chemical change during the molding process and cannot be effectively reshaped and recycled—except by grinding and using them as fillers for the construction industry.
Today, a world without plastic is almost unimaginable, even more so due to the COVID-19 pandemic. But while durability and inexpensiveness, two of plastic’s greatest assets, have contributed to make it the workhorse material of our modern society, they have also become its greatest curse. The associated linear economy approach has resulted in almost 90% of plastic products being used only once and then discarded, creating today’s global environmental and sustainability crisis. As discussed, from the inception of the industry more than 6.3 billion tons of plastic waste have been produced worldwide;46 of this waste about 79% ended in landfills or the environment, 12% was incinerated, and only about 9% was recycled.47
Up to now, the common strategy to combat the escalating plastic pandemic has been a “3Rs” approach: reduce, reuse and recycle, but success requires transitioning to a truly circular, globally integrated and decarbonized plastic economy—where products are 100% recyclable, used for as long as possible, and waste is minimized.48
Recycling
In order to understand the life cycle49 of plastics, it is important to remember that not all plastics are equal. As discussed, plastics not only have different chemical and physical properties, but also have different utilization lives. Furthermore, some are products by themselves (like water bottles) while others are parts of more complex products. For this reason, post-consumer plastic waste is usually very inhomogeneous and comprises a wide range of material, shape, color and size types requiring separation—a costly, time and energy intensive process which often leads to lower quality materials. For example, even something apparently as simple as color presents a challenge. Plastics of the same chemical composition, but different colors, are very difficult to separate using existing industrial sorting processes. Hence plastics of various colors tend to be recycled together, limiting the choice of the final product color to black. Finally, current recycling technologies cannot be applied to all polymeric materials, as is the case for thermosets. All these factors are further exacerbated by the lack of global standards and coordination across the value chain, which has allowed the proliferation of different materials, labelling, collection schemes, sorting and reprocessing systems, all of which hinder recyclability.
From an economic point of view, recycling is dictated by market demand, price, and regulatory considerations, and involves all stakeholders along the value chain. Consumers play a critical role since the way plastic products are used and disposed of has a significant impact on their quality and value post-use.
Today, mechanical recycling50 or “chop-and-wash” is the only widely adopted technology for the large-scale treatment of plastic solid waste. It is a process in which plastics are sorted by material type, grounded, washed, and then dried to produce recycled flakes, but due to the high temperatures used, progressive material deterioration becomes an issue. The resulting flakes are then usually blended with virgin plastic of the same type to produce materials with suitable properties for manufacturing. In general, the higher the temperature a polymer can withstand without losing its properties, the higher the quality it will have as a recycled material, but due to progressive deterioration plastics cannot be recycled infinitely.
Alternative approaches to mechanical recycling include chemical recycling,51 a process encompassing various technologies such as pyrolysis5253 with a high tolerance to mixed and contaminated plastic waste streams and yielding materials of higher quality than those obtainable through simple mechanical recycling. The goal of chemical recycling is to break the long polymer chains down into their original building blocks (or monomers) which can then be used as feedstocks for new plastics, fuels, waxes, or other valuable products. But this approach is much more capital-intensive than mechanical recycling since it requires complex industrial processing equipment, and hence overall economics tend to be less favorable compared to the production of new virgin plastic. As a result, this route is not yet followed at an industrial scale globally.54
Incineration is sometimes also listed as a recycling option since proponents claim that it allows for energy recovery in the form of heat.While the process might be convenient for the treatment of mixed waste because it avoids the need for sorting, it does not allow for recovery and reuse. Furthermore, since carbon is a major building block of plastic, simple incineration is a source of greenhouse gas emissions and potentially other hazardous substances. Overall, the use of virgin plastic results in two different streams of CO2 emissions. The first derives from the energy consumption associated with the production process itself—generating on average 2.5 tons of CO2 per ton of plastic. The second is due to the emissions deriving from the eventual end-of-life incineration and/or decomposition of plastic—equivalent to about 2.7 tons of CO2 per ton of plastic.55 For these reasons, in our opinion, incineration is an example of an old linear economy approach and not a recycling option.
The Sustainable Future of Plastic
Since today’s plastics can stay in the environment for hundreds of years and even when degraded, they never truly leave our ecosystems, but remain present as smaller pieces or particles, a new paradigm is needed. Achieving a long-term sustainable future for plastic will require the transition to truly circular value chains and an increased focus on plastic composition through both the smarter design of polymers to improve recyclability and the development of bio-based alternatives—a process that will require much deeper integration along value chains and the active participation of all stakeholders from product-designers, to consumers, to waste collectors, recycling workers, and policy makers.
From an innovation perspective, on one hand polymer designs that enable selective degradation56 to building block molecules will be key in addressing how to effectively separate and recycle polymers from different types of plastic blends. On the other, biobased plastic alternatives in which polymers are made from natural renewable materials such as plants, instead of fossil fuels will require the development of a portfolio of carbon-neutral building block molecules without impacting land use. In 2017, global land requirements to grow the needed feedstocks for biobased plastic demand (about 1% of total global plastics production) were estimated to be about 0.02% of arable lands. If all fossil fuel-based plastics were to be produced from biomass, it would require about 5% of overall worldwide biomass production.57 In other words, biobased materials could become the eco-friendly alternative to conventional plastic materials by not only reducing fossil fuels consumption, but also providing the unique potential of carbon neutrality, provided that the land required for bioplastics would not compete with food production. At the same time, like traditional plastic, biobased polymers will need to be able to withstand high temperatures to avoid material degradation and thus enhance recyclability.58
A plastic material can be identified as a bioplastic if it is either biobased, biodegradable, or both (see Figure 3). Biodegradation is a chemical process by which microorganisms present in the environment convert materials into water, carbon dioxide, and compost. It should be noted that being biobased does not automatically equate into being also biodegradable—some 100 percent biobased plastics may not be biodegradable while some 100 percent petroleum-based plastics may. The prefix ‘bio’ should also not be misleading by suggesting that a bioplastic material has a smaller environmental impact than the equivalent petroleum-based one. This is not always the case as it is a function of the production and disposal processes employed; hence full life cycle analyses of substitute materials will be critical to determine their true sustainability.59
Bioplastics obviously also need to replicate the properties of traditional plastic and for some products they already do, but they tend to be more expensive on a weight basis,60 hindering adoption. However, innovation and economies of scale are driving costs down. It should also be noted that while production costs of fossil-based plastics are a function of oil prices and thus fluctuate overtime, the costs of bio-based plastics depend on biomass prices that are thus more stable. Today the two most commonly used bioplastics are PLA (or polylactic acid) derived from corn starch or sugar cane and PHA (or polyhydroxyalkanoate) produced by microorganisms from organic materials such as food waste, crop residues, and waste oils.
PLA is by far the most commercially developed and it is used in disposable utensils, straws, bottles, packaging,61 and for 3D printing. However, it has a low melting point, so it cannot be used with hot foods or liquids. PHA, on the other hand, is mainly used for packaging and medical applications—like sutures and cardiovascular patches.
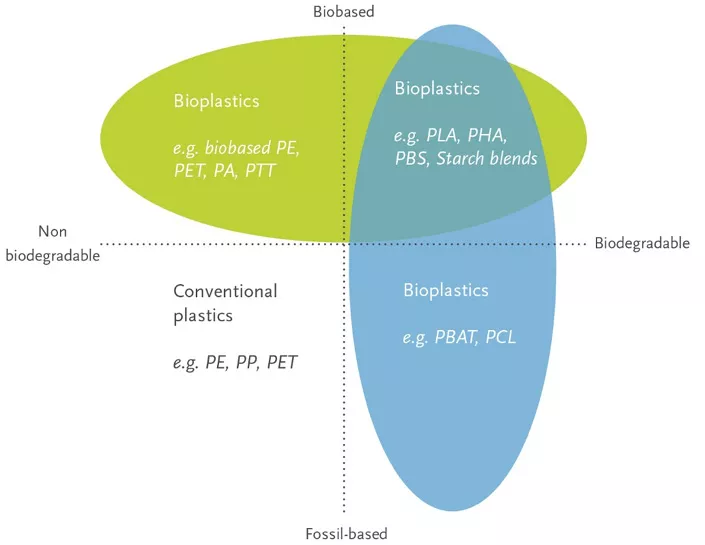
Figure 3: What are bioplastics? (European Bioplastics 2020)
While biodegradable bioplastics are often marketed as compostable, in practice it is not that simple. PLA, for example, does not naturally degrade in landfills or home compost heaps on its own in consequential timeframes, but needs to be sent to industrial composting facilities where it can be heated to the temperatures required for microbes to break it down. Although the number of specialized facilities is growing, the lack of compatibility with existing processing and recycling systems to avoid cross contamination with traditional plastics needs to be significantly improved.62
In summary, bioplastics have the potential to alleviate some of the long-term environmental and sustainability challenges of petroleum-based plastics, from potentially carbon-neutral manufacturing processes to the ability to biodegrade. However, bioplastics are currently more expensive than traditional plastics, and might not be as eco-friendly as they seem.
To guarantee long-term business sustainability, companies around the world have begun to invest in these innovative efforts. One example is Versalis, the petrochemical subsidiary of the Italian energy major Eni, for which circularity is a stated competitive lever driving an innovation strategy based on “three pillars”: eco-design to maximize resource efficiency, recycling and reuse technologies, and bio-based alternatives to conventional feedstocks.63
Conclusions and Recommendations
Plastic has become so omnipresent in our daily lives that it is simply impossible to imagine a world without it. However, lack of post-use considerations, high costs of recycling, together with a significant carbon footprint have turned plastic into the victim of its own success and created a global sustainability and environmental crisis. This is an issue even more so during the on-going COVID-19 pandemic, which has significantly increased plastic demand in response to the virus. While governments and the general public in developed nations are increasingly conscious of these issues, in emerging and developing economies awareness is hindered by the challenges of rapid population growth and urban governance.
Up to now, the strategy to address the challenges associated with plastic production and use has been mainly based on a “3Rs” approach: reduce, reuse, and recycle. Although any measure to curb the environmental impacts of plastic is a step in the right direction, a systematic change is needed. The transition to a sustainable future for plastic must encompass every aspect of the value chain, from production to consumption to disposal, and be based on a circular economy approach to ensure meaningful and overall positive effects. This will require not only a shift to fossil-free feedstocks and energy to produce carbon-neutral building blocks, but also the development of new plastic designs focusing on both material functionality and recyclability.
Going forward, the industry must continue to move away from single-use plastic and focus on products that can be reused multiple times thus reducing demand for plastic altogether. But this approach alone will not be enough, considering how ubiquitous single use-plastic isin so many sectors—and vital for some.
Second, the challenges of mixed plastic waste, deteriorating material properties, together with the low cost of virgin plastic are currently key issues in creating demand for recycled plastic and will need to be addressed globally both from a technological and policy point of view. In the latter case, mandates on recycling targets and percentages of post-consumer recycled (PCR) materials in new products will be key. At the same time, policymakers will need to develop incentives to rationalize post use plastic value chains which today are highlyfragmented and localized.
From an innovation point of view, three research pathways show significant potential to advance plastic recycling practices: improving chemical recycling efficiency and selectivity, minimizing sorting needs through smarter designs, and expanding recycling beyond thermoplastics. Furthermore, as the plastic industry becomes more circular it will also be important to address the recycling and composting requirements of bioplastic—which are different from those of petroleum-based plastic.
Finally, decarbonization of plastic production will require both switching to low carbon energy sources, such as renewable energy or green hydrogen, and using bio-feedstocks to develop carbon-neutral alternatives to traditional plastic. Detailed life-cycle analyses of each new route and/or market will be key to identify cross-cutting benefits and costs and hence to ensure overall sustainability. To build demand for bioplastic, similar to renewable energy standards, policymakers could introduce “renewable plastic standards” requiring the private sector to provide customers with a stated minimum share bioplastic with respect to overall production, starting from higher value products. This would require some level of international cooperation because bioplastics are more expensive and could not be competitive in commodity markets without appropriate trade measures.
From this perspective, we would recommend an approach to plastic focusing on innovation that includes the following elements:
- Increase recyclability of plastics. Redesign products to be easily recycled and reused. For example, instead of combining many types of plastic in one product, which makes recycling more difficult and expensive, redesign production so that manufactured goods are made of only one type of plastic. Limit the number of specialized plastics and of additives. Introduce extended producer responsibility to create incentives for plastic products to be more easily recyclable.
- Enhance recycling. Improve and harmonize existing collection and recycling systems. Build an infrastructure tailored to recycling bioplastic, for example by increasing access to industrial composting plants.Mandaterecycling targets and percentages of recycled materials in new products. Incentivize recycling for consumers.
- Decarbonize production. Switch to low carbon energy sources such as renewable energy or green hydrogen, while substituting petroleum-based plastics with more sustainable materials such as bioplastics. Conduct life cycle analyses on new products to ensure their overall sustainability, rather than just one aspect of their use. Build demand for bioplastic through policies, like renewable plastic standards, starting from high value products.
- Development of New Public Private Partnerships. Foster government support for research and development—both through investment vehicles and private public partnerships. Align incentives, for example by requiring producers to address the negative externalities of plastic waste, such as ocean clean-up. Develop global partnerships that combine technical and regulatory factors to harmonize value chains.
- A concerted approach to demystify the plastic sector and ensure that local communities and the public at large have appropriate appreciation for the critical role they play. Educate consumers on plastic lifecycle and incentivize sustainable practices, as the way plastic products are used and disposed of has a significant impact on their value and quality post-use.
To date, technological factors, economic considerations, but also consumers’ behavior have hindered the sustainable use of plastic and created a global environmental crisis. New geopolitical forces—such as the challenges of development and climate change—are reshaping the playing field and stakeholders around the world will need to decide their role in this transition.
De Blasio, Nicola and Phoebe Fallon. “Avoiding a Plastic Pandemic: The Future of Sustainability in a Post COVID-19 World.” January 2021
- UN Environment (2018), “Our Planet Is Drowning in Plastic Pollution”, https://www.unenvironment.org/interactive/beat-plastic-pollution/, accessed September 18, 2020.
- Recycling refers to the reprocessing of used materials into new products.
- Ellen MacArthur Foundation (2016), “The New Plastics Economy: Rethinking the Future of Plastics” https://www.ellenmacarthurfoundation.org/assets/downloads/EllenMacArthurFoundation_TheNewPlasticsEconomy_Pages.pdf, accessed August 10, 2020.
- Reddy, S. and Lau, W. (2020), “Breaking the Plastic Wave: Top Findings for Preventing Plastic Pollution” Pew Research Center https://www.pewtrusts.org/en/research-and-analysis/articles/2020/07/23/breaking-the-plastic-wave-top-findings, accessed July 23, 2020.
- Ellen MacArthur Foundation (2016), “The New Plastics Economy: Rethinking the Future of Plastics” https://www.ellenmacarthurfoundation.org/assets/downloads/EllenMacArthurFoundation_TheNewPlasticsEconomy_Pages.pdf, accessed August 10, 2020.
- Romera-Castillo, C., Pinto, M., Langer, T. M., Álvarez-Salgado, X. A. & Herndl, G. J. (2018), “Dissolved organic carbon leaching from plastics stimulates microbial activity in the ocean” Nat. Commun. 9, 1430.
- Center for International Environmental Law (2019), “Plastic & Climate: The Hidden Costs of a Plastic Planet” https://www.ciel.org/wp-content/uploads/2019/05/Plastic-and-Climate-FINAL-2019.pdf.
- IEA (2020), “Global CO2 Emissions in 2019” https://www.iea.org/articles/global-co2-emissions-in-2019.
- Wautelet, T. (2018), “Exploring the role of independent retailers in the circular economy: a case study approach” 10.13140/RG.2.2.17085.15847.
- Tenenbaum, L. (2020), “The Amount of Plastic Waste is Surging Because of the Coronavirus Pandemic” Forbes, https://www.forbes.com/sites/lauratenenbaum/2020/04/25/plastic-waste-du… accessed July 27, 2020.
- Leonard, G. and Mallos, N. (2020), “What We Know- and Don’t Know- About Plastics and the Coronavirus” Ocean Conservancy, https://oceanconservancy.org/blog/2020/04/16/know-dont-know-plastics-coronavirus-pandemic/ accessed July 27, 2020.
- Chaudhuri, S. (2020), “How Coronavirus Complicated the Quest for a Greener Plastic Bottle” Wall Street Journal, https://www.wsj.com/articles/how-the-coronavirus-complicated-the-quest-for-a-greener-plastic-bottle-11593005945 accessed July 27, 2020.
- Parker, L. (2018), “Straw Wars: The Fight to Rid the Oceans of Plastic” National Geographichttps://www.nationalgeographic.com/news/2017/04/plastic-straws-ocean-trash-environment/, accessed July 6, 2020.
- National Conference of State Legislatures (2020), “State Plastic and Paper Ban Legislation” https://www.ncsl.org/research/environment-and-natural-resources/plastic-bag-legislation.aspx, accessed July 10, 2020.
- Chaudhuri, S. (2020), “How Coronavirus Complicated the Quest for a Greener Plastic Bottle” Wall Street Journalhttps://www.wsj.com/articles/how-the-coronavirus-complicated-the-quest-for-a-greener-plastic-bottle-11593005945, accessed July 27, 2020.
- Environmental Protection Agency (2015), “U.S. EPA Sustainable Materials Management Program Strategic Plan”, https://www.epa.gov/sites/production/files/2016-03/documents/smm_strategic_plan_october_2015.pdf, accessed July 2, 2020.
- European Commission (2015), “Closing the loop - An EU action plan for the Circular Economy” https://eur-lex.europa.eu/legal-content/EN/TXT/?uri=CELEX:52015DC0614 , accessed October 20, 2020.
- European Commission (2018), “First Circular Economy Action Plan” https://ec.europa.eu/environment/circular-economy/first_circular_economy_action_plan.html, accessed July 8, 2020.
- European Commission (2018), “A European Strategy for Plastics in a Circular Economy, https://eur-lex.europa.eu/resource.html?uri=cellar:2df5d1d2-fac7-11e7-b8f5-01aa75ed71a1.0001.02/DOC_1&format=PDF, accessed July 8, 2020.
- UN (2015), “2030 Agenda for Sustainable Development” https://sustainabledevelopment.un.org/post2015/transformingourworld, accessed October 10, 2020.
- Independent Group of Scientists appointed by the Secretary-General (2019), “Global Sustainable Development Report 2019: The Future is Now—Science for Achieving Sustainable Development” https://sustainabledevelopment.un.org/content/documents/24797GSDR_report_2019.pdf, accessed July 8, 2020.
- Ellen MacArthur Foundation (2017), “The New Plastics Economy Catalyzing Action” https://www.newplasticseconomy.org/assets/doc/New-Plastics-Economy_Catalysing-Action_13-1-17.pdf, accessed July 12, 2020.
- Amcor (2019), “Sustainability Review 2019” https://assets.ctfassets.net/f7tuyt85vtoa/4MJVhRWWstldLyyNHt1dFY/93a8af747a3b66089efbbed118f42af7/Sustainability_Review_V11_AW_Digital.pdf, accessed July 16, 2020.
- Clifford, T. (2020), “Hospital supplier Owens & Minor is expanding capacity to meet personal protective equipment demands” CNBC https://www.cnbc.com/2020/03/27/hospital-supplier-owens-minor-expanding-capacity-to-meet-ppe-demands.html, accessed August 16, 2020.
- WHO (2020) “Shortage of personal protective equipment endangering health workers worldwide” https://www.who.int/news/item/03-03-2020-shortage-of-personal-protective-equipment-endangering-health-workers-worldwide, accessed Sept. 28, 2020.
- Henneberry, B. (2020), “How Surgical Masks are Made” Thomas Publishing Company https://www.thomasnet.com/articles/other/how-surgical-masks-are-made/, accessed August 18, 2020.
- Paxton, V. (2010), “Medical Gloves—Types and Uses” AllegroMedical https://blog.allegromedical.com/medical-glove-types-latex-vinyl-and-nitrile-exam-and-surgical-gloves-buyers-guide-1534.html, accessed August 18, 2020.
- Edwards, E. (2020) “How are Goggles Made?” Thomas Publishing Company https://www.thomasnet.com/articles/plant-facility-equipment/how-are-goggles-made/, accessed August 18, 2020
- Center for Disease Control and Prevention (2020), “Optimizing Supply of PPE and Other Equipment During Shortages” https://www.cdc.gov/coronavirus/2019-ncov/hcp/ppe-strategy/isolation-gowns.html, accessed August 18, 2020.
- Howard, J., Liptak, K. and Valencia, N. (2020), “CDC’s draft guidance for reopening amid coronavirus includes spaced-out seating in schools, disposable menus in restaurants” CNN https://www.cnn.com/2020/04/30/health/coronavirus-usa-cdc-reopening-bn-wellness/index.html, accessed August 18, 2020.
- Peszko, G. (2020), “Plastics: The Coronavirus could reset the Clock” World Bank, https://blogs.worldbank.org/voices/plastics-coronavirus-could-reset-clock, accessed August 18, 2020.
- Chasan, E. (2020), “The Oil Crash Created a Recycled Plastic Trap” Bloomberg https://www.bloomberg.com/news/articles/2020-05-06/oil-crash-means-single-use-plastic-is-back-as-recycling-struggles, accessed August 21, 2020.
- Consumer Brand Association (2020), https://consumerbrandsassociation.org/sustainability/recycling-policy-platform/, accessed August 18, 2020.
- Chaudhuri, S. (2020), “How Coronavirus Complicated the Quest for a Greener Plastic Bottle” Wall Street Journal https://www.wsj.com/articles/how-the-coronavirus-complicated-the-quest-for-a-greener-plastic-bottle-11593005945, accessed August 16 2020.
- Fletcher, C. (2020), “How is Used PPE Disposed of During the Coronavirus Pandemic” Global Biodefense https://globalbiodefense.com/2020/05/12/how-is-used-ppe-disposed-of-during-the-coronavirus-pandemic/, accessed August 21, 2020.
- Maiden, T., Patton, B., Balaster, M. and Schmoll, E. (2020), “Novel Coronavirus—How employers should dispose of personal protective equipment” ReedSmithhttps://www.ehslawinsights.com/2020/04/novel-coronavirus-how-employers-should-dispose-of-personal-protective-equipment/, accessed August 21, 2020.
- Chaudhuri, S. (2020), “How Coronavirus Complicated the Quest for a Greener Plastic Bottle” Wall Street Journalhttps://www.wsj.com/articles/how-the-coronavirus-complicated-the-quest-for-a-greener-plastic-bottle-11593005945, accessed August 16, 2020.
- Rosengren, C., Crunden, E.A. (2020), “How coronavirus is disrupting US waste and recycling” WasteDivehttps://www.wastedive.com/news/coronavirus-us-waste-recycling-disruption-tracker/574324/, accessed August 16, 2020.
- Hahladakis, J., Velis, C., Weber, R., Iacovidou, E. and Purnel, P. (2018), “An overview of chemical additives present in plastics: Migration, release, fate and environmental impact during their use, disposal and recycling” Journal Haz. Mat. V344, 179-199 https://doi.org/10.1016/j.jhazmat.2017.10.014.
- Plastics Industry Association (2020), “History of Plastics”https://www.plasticsindustry.org/history-plastics, accessed Sep. 1, 2020.
- Nova Institute (2020), “Bio-based Building Blocks and Polymers—Global Capacities, Production and Trends 2019-2024” http://nova-institute.eu/press/?id=164 accessed Sept. 1, 2020.
- Plastic Europe (2019), “Plastics - the Facts 2019” https://www.plasticseurope.org/en/resources/market-data accessed Sept. 1, 2020.
- Ibid.
- The word polymer derives from the Greek “polus” meaning many, and “meros” meaning parts or units. A polymer can be thought of as a very long chain in which each link is a monomer (single unit). Although most polymers contain only carbon and hydrogen, some may also contain oxygen, chlorine, fluorine, nitrogen, silicon, phosphorus, and sulfur. While most plastics are based on the carbon atom, silicones are based on silicon.
- Thermoplastics mainly constitute the plastic objects of everyday life. Thermosets tend to be more specialty materials and resins and are converted to chemically cross-linked polymers, during the molding process, creating a three-dimensional network.
- Ellen MacArthur Foundation (2016), “The New Plastics Economy: Rethinking the Future of Plastics” https://www.ellenmacarthurfoundation.org/publications/thenew-plastics-economy-rethinking-the-future-ofplastics accessed Aug. 10, 2020.
- Geyer, R., Jambeck, J., and Law, K. (2017), “Production, use, and fate of all plastics ever made” Sci. Adv. 3, e1700782 DOI: 10.1126/sciadv.1700782.
- European Commission (2018), “A European Strategy for Plastics in a Circular Economy” https://ec.europa.eu/environment/circular-economy/pdf/plastics-strategy-brochure.pdf accessed Sept. 2, 2020.
- The life cycle of a product incorporates all phases of its production and use, from resource extraction to end-of-life disposal or recycling. Energy consumption and environmental impacts in all phases contribute to its total energy intensity, use intensity, and carbon intensity.
- Grigore, M. (2017), “Methods of Recycling, Properties and Applications of Recycled Thermoplastic Polymers” Recycling 2, 24, https://doi:10.3390/recycling2040024.
- Also called advanced recycling or “back to monomer” recycling.
- A thermolysis process to selectively produce gases, fuels, or waxes using catalysts in the absence of oxygen to prevent combustion.
- Aguado, R., Olazar, M., San José, M., and Bilbao, J. (2002), “Wax Formation in the Pyrolysis of Polyolefins in a Conical Spouted Bed Reactor” Energy Fuels 16, 1429.
- Rahimi, A. and García, J. M. (2017), “Chemical recycling of waste plastics for new materials production” Nature Reviews Chemistry, Vol. 1, 1-11, https://doi.org/10.1038/s41570-017-0046.
- Material Economics (2018), The circular economy—a powerful force for climate mitigation: https://materialeconomics.com/publications/the-circular-economy-a-powerful-force-for-climate-mitigation-1 accessed Oct. 15, 2020.
- Selective degradation refers to the ability of controlling the course of polymers degradation and it is a key issue in designing degradable materials.
- Van den Oever, M., Molenveld, K., van der Zee, M., and Bos. H. (2017), “Bio-based and biodegradable plastics—Facts and Figures” Wageningen Food & Biobased Research, Report 1722, doi: 10.18174/408350.
- Hatti-Kaul, R., Nilsson, L., Zhang, B., Rehnberg, N. and Lundmark, S. (2019), “Designing Biobased Recyclable Polymers for Plastics” Trends in Biotechnology 38(1), 50-67 doi: 10.1016/j.tibtech.2019.04.011.
- Cho, R. (2017), “The Truth About Bioplastics” Earth Institute Columbia University https://blogs.ei.columbia.edu/2017/12/13/the-truth-about-bioplastics/, accessed August 9, 2020.
- Van den Oever, M., Molenveld, K., van der Zee, M., and Bos. H. (2017), “Bio-based and biodegradable plastics—Facts and Figures” Wageningen Food & Biobased Research, Report 1722, doi: 10.18174/408350.
- Gibbens, S. (2018), “What you need to know about plant-based bioplastics” National Geographic https://www.nationalgeographic.com/environment/2018/11/are-bioplastics-…, accessed August 9, 2020.
- Peters, A. (2019), “Will Compostable Packaging Ever be able to Solve our Waste Problem?” Fast Company, https://www.fastcompany.com/90393297/will-compostable-packaging-ever-be-able-to-solve-our-waste-problem, accessed October 3rd, 2020.
- Eni Versalis (2020), “Circular Economy” https://www.versalis.eni.com/irj/portal/anonymous?guest_user=anon_en&NavigationTarget=ROLES://portal_content/z_eni_ve_fl_versalis/z_eni_ve_fl_roles/z_eni_ve_rl_gues_versalis/EconomiaCircolare, accessed November 20, 2020.