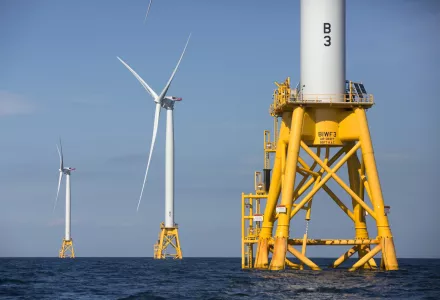
In the last decade, many states set ambitious goals to decarbonize their energy portfolios. Seven states along the Atlantic coast are pursuing 100% clean electricity targets, while others have adopted stringent emissions reduction targets.1 Realizing these goals requires scaling wind, solar, and other renewable resource electricity generation at rates three to four times recent levels. If wind is to play a significant role in decarbonizing the electricity sector in the densely populated northeast section of the United States, a substantial proportion of the investment must occur offshore, as onshore wind speeds and available land area are limited. Some states have taken an additional step by establishing specific offshore wind targets. For example, New York's 2019 Climate Leadership and Community Protection Act contains a goal of 9,000 MW of offshore wind by 2035.2 This policy brief examines the economic and technical feasibility of substantial wind generation development along the Eastern Seaboard.
The national offshore wind (OSW) resource base is enormous, but the United States has been slow to tap into this resource. While Europe has invested in OSW over the past three decades, OSW is only now beginning to gain traction in the United States. As of 2021, the United States has installed only 42 MW of offshore wind compared to more than 22,000 MW in Europe.3 The economic benefits of investing in this resource could be significant. The U.S. Department of Energy (DOE) and Department of the Interior (DOI) estimate that capturing 86 gigawatts (GW) of OSW by 2050 could generate substantial economic and environmental benefits, including a 1.8% decrease in greenhouse gas emissions, a 5% decrease in power sector water consumption, approximately 160,000 domestic jobs, and avoided public health damages as a result of reduced air pollution.4 Significant barriers to achieving these benefits include OSW's high costs relative to other energy sources, a lack of development of the wind energy market and its supply chain (e.g. OSW construction knowledge), and concerns about environmental impacts both onshore and offshore.
Policy support for offshore wind is increasing dramatically, driven both by ambitious decarbonization targets and the inability to site incremental onshore wind. The Biden administration announced an offshore wind target of 30 GW by 2030.5 Several OSW projects expect to begin construction in the early 2020s, promising to boost the United States’ total operational capacity to over 9,100 MW across 14 projects by 2026, if all projects are completed as planned.6
If decreases in levelized costs, forecasted by the industry, are realized, OSW is on track to become cost-competitive with other energy resources within the decade. While not the only useful measure for a project, levelized cost of energy (LCOE) is a helpful metric because it considers the present value of costs across a project's lifetime against the amount of energy produced. LCOE enables comparisons across projects regardless of capacity, energy source, and capital costs. LCOE is measured in dollars per megawatt hour or dollars per kilowatt hour.7
A 2016 National Renewable Energy Laboratory (NREL) analysis estimated that East Coast projects with 2022 commercial operation dates will have LCOEs between $95/MWh and $250/MWh, with the large range “reflect[ing] the variation in geospatial characteristics among U.S. offshore wind site conditions.”8 NREL estimated that this range shifts to between $80/MWh and $170/MWh by 2027. The costs for projects in the North Atlantic tend to be lower than those for projects in the South Atlantic, primarily due to lower wind speeds.9 NREL notes that "the lowest-cost sites are characterized by annual average wind speeds ranging from 9 meters per second (m/s) - 10 m/s (at hub heights of 100 m), with water depths less than 20 m, and located less than 50 km from shore."10
In this policy brief, we present an estimate of the baseline costs and benefits of OSW deployment in the eastern United States absent federal tax incentives or projected future technology improvements to advance the understanding of the “raw cost” for a hypothetical offshore wind project as of the end of 2020. Technology and exact costs may change, but this analysis can serve as a starting template for evaluating the financial viability of OSW projects.
Our analysis results in a range of between 11.7¢ per kWh and 14.3¢ per kWh (LCOE) for a hypothetical 800 MW OSW project becoming operational in 2026, with a 30-year operational lifespan and a 10% contingency to account for uncertainty.i This theoretical project is not tied to a specific state or region of the East Coast (e.g., Northeast, mid-Atlantic), as it is intended to encompass a generic Eastern baseline. The exact LCOE will vary due to a particular state or region's circumstances. The LCOE will also be influenced by natural factors that fluctuate along the coast, such as wind speed. For this analysis, we assume an average wind speed of 8.5 m/s at a 100-meter turbine height, 50 miles to onshore grid connection, and an average capacity factor of 46%. Actual capacity factors are expected to be lower in the South Atlantic and slightly higher in the North Atlantic.11
To evaluate the costs of a typical OSW project under development in the early 2020s, we model a hypothetical 800 MW project with a 2026 commercial operation date (COD). These inputs are comparable to several states' plans and ongoing projects. For example, Dominion Energy's planned 2,600 MW project in Virginia, slated to begin construction in 2024, is the largest U.S. offshore projected announced in North America.12 That project would be constructed across three 880 MW tranches, similar to the 800 MW tranche considered here.13
We assume that projects will use 12 MW turbines, which are the best available technology. As of late 2020, General Electric (GE) was the only turbine manufacturer offering a 12 MW turbine design—its Haliade-X 12 MW turbine. In September 2019, Danish offshore wind developer Ørsted announced its intent to use the 12 MW GE turbines for the Skipjack Wind project in Maryland (expected COD: 2022) and the Ocean Wind project in New Jersey (expected COD: 2024).14 Larger turbines can contribute to a higher capacity factor as longer blades can generate energy under a broader range of wind conditions.15
GE's projected capacity factor for the Haliade-X 12 MW is 63%, based on wind conditions in the German North Sea.16 This represents a 50% increase in efficiency over GE's 8 MW turbine.17 However, wind speeds in the North Sea are greater than in most areas of the eastern U.S., thus it is expected that capacity factors for U.S. applications will be lower. To account for the differences in wind speed along the coast, we used an estimated capacity factor of 46%. Estimates of capacity factors have a substantial impact on cost estimates; a ten percentage-point increase in capacity factor will decrease costs by approximately 1.2¢ per kWh or approximately 10%.
Curtailment—which occurs when energy is produced but ultimately not used by the grid—also affects cost estimates. We assume a curtailment of 3% to capture generation reductions from grid operation constraints, such as limited transmission network infrastructure, resulting in periodic congestion.18 The curtailment value is also subject to variability based on system conditions, including the quantity of generation and transmission availability. Curtailment levels will vary over time and should be monitored and revisited.
The expected timespan of a hypothetical project is 34 years: two years of construction, 30 years of operation, and two years of decommissioning at the end of the project's life.19 We consider costs across each phase, using a 7% discount rate.
Gathering information about OSW financing costs and cost of capital is “often challenging given the confidential nature of project finance data.”20 Here, we assume 65% debt, a debt term of 30 years, and an interest rate of 3.7%.21 The estimated LCOE includes a 10% contingency. Many existing renewable investments in the United States include a significant percent of tax equity. Project developers receive credits from the federal government, which they sell to entities with larger tax liabilities to generate capital. As the amount of offshore wind increases, this market may diminish and the margins may shrink. Therefore, private equity costs could rise, as the tax equity market decreases.
Economic outcomes like jobs, gross domestic product, labor income, and tax revenue are not considered in this analysis. However, these factors often account for much of the political support for offshore wind projects, even though many of them are simply economic transfers and are not real economic benefits.
Variables that could influence LCOE
The actual LCOE will depend on several external factors that influence project cost, including the availability of tax incentives, the number of projects already operational, technology improvements, and transmission infrastructure.
Tax Incentives: In December 2020, Congress extended and increased the federal investment tax credit (ITC) for OSW as part of the $900 billion COVID-19 relief package.22 Projects that begin construction through 2025 will be eligible for an ITC of up to 30% of investment costs.23 In concert with the ITC increase and extension, the Internal Revenue Service (IRS) released Notice 2021-05, which extends the Continuity Safe Harbor for OSW projects that begin construction by 2025 and enter service up to 10 years after construction commences, allowing those projects to receive the full value of the ITC.24Thus, projects that start construction in 2025 and begin operation in 2035 would be eligible. Congress's COVID-19 relief package also extended a production tax credit (PTC) for wind projects beginning construction through 2021. Eligible OSW projects may select either the ITC or the PTC.25 Our initial estimates assume no ITC or PTC to provide the most "bare-bones," conservative cost estimate. With the addition of tax credits, OSW projects can deliver energy at a lower cost, either in the form of lower wholesale prices, higher cash flows, or lower capital costs. In all three cases, consumers should reap benefits. The size of the benefit will be affected by the continuing availability of tax equity. As of November 2021, Congress was considering legislation that would increase the production tax credit further.
Technology and Efficiency: Even in the absence of a tax credit, OSW project costs are predicted to decrease over time. Cost reduction opportunities for OSW include innovations in turbine design, increased size of turbines, improvements in grid connection technology, standardization of parts, and economies of scale. The U.S. Offshore Wind Market and Economic Analysis projected that capital and operations and maintenance costs for new OSW projects will decline 2.8% year over year between 2020 and 2022, 2.1% year over year between 2023 and 2027, and 1.5% between 2027 and 2028.26 Under these projections, a project that costs $100 million in 2020 will cost $83.7 million in 2028, assuming constant dollars. Factors such as workforce training and improved technical capability (e.g., existence of U.S. flagged vessels capable of installing turbines in compliance with the Jones Act) will further reduce costs.
Transmission Infrastructure: The type of offshore transmission line selected has an impact on project costs. This analysis estimates AC (alternating current) cables stretching 50 miles to the onshore connection point and associated transformer stations. While AC transmission is cheaper than DC (direct current), it transmits less energy and is better suited for shorter distances between turbine arrays and onshore transmission sub-stations.27 DC transmission is more efficient, as it has a higher capacity and can reach further distances from shore. Onshore transmission infrastructure also affects costs. Inserting new resources into existing grid capacity may require substantial onshore infrastructure upgrades. Transmission improvements will increase prices for the consumer. How much is uncertain and will depend on both demand and on the regulatory treatment of rates.
Carbon Pricing: A future policy placing a price on carbon would increase the cost of power from fossil-fueled electricity generators, which should improve the cost competitiveness of renewable options. Carbon pricing can internalize the negative externalities associated with fossil fuel generation regarding public health and climate change impacts. Carbon pricing will not reduce the cost of offshore wind, but will increase the cost of alternatives, such as natural-gas-fired power.
In summary, the investment and production tax credits together with the projected cost reductions from technical improvements will reduce the cost of offshore wind to levels in the range of 7.5¢ per kWh to 10¢ per kWh.ii Increased transmission costs and delays in permitting and siting will raise these numbers, hence, the actual increases will be site dependent.
Challenges
Opposition to land-based wind projects and inadequate sunlight during the winter months, as well as the daytime limitations of solar production absent adequate energy storage capacity, makes offshore wind one of the best options to complement existing renewable energy production options and meet aggressive goals to decarbonize the electricity sector. However, there are three caveats that energy planners should keep in mind. First, developing large amounts of wind offshore with capacity factors approximately 50% of natural-gas-fired plants creates a system problem when the wind is not blowing. To make this problem more challenging, the wind resource is less predictable and can vary year to year and month to month. What happens to a system which has 40% of its generating capacity offshore, when the wind resource for one month is 30% of what it had been the previous month?
Perhaps longer-term storage options will have emerged or the economics of natural gas facilities with carbon capture and sequestration options will be economically feasible and available. But the greater the investment in offshore wind, with its comparatively low-capacity factors, the larger will be the challenge facing the local grid operators—a challenge that is sometimes forgotten in the heat of the political debate.
Second, the reduction in emissions from offshore wind is a function of being able to retire or curtail generation from fossil-fueled electricity generators. The ability to guarantee such retirements is limited by the availability of transmission to move power to all of the loads now served by the fossil-fueled generators. Offshore wind generated off the coast of one state may end up being sent to a neighboring state. At a minimum, states will need to build institutional mechanisms to work together to ensure that the transmission network is available to optimize the use of large amounts of offshore-wind-generated power that is likely to be in place 10 years from now.
Finally, the optimal regulatory system, including tariff structures, for the existing system is not likely to be optimal for a system that relies heavily on intermittent generation options. Without strong incentives to stimulate optimal investments and operating practices, the potential benefits of offshore wind may not be fully realized.
[i] Estimates based on best available information at the end of 2020.
[ii] These estimates are based on the best available information as of June 2021.
[1] Natural Resources Defense Council (NRDC). “Race to 100% Clean.” Clean Energy, 2 Dec. 2020.
[2] New York State Senate Bill S6599.
[3] Wind Europe. “Offshore Wind in Europe – Key Trends and Statistics 2019.” WindEurope, 6 Feb. 2020.
[4] U.S. Department of Energy and U.S. Department of the Interior. National Offshore Wind Strategy: Facilitating the Development of the Offshore Wind Industry in the United States, 2016.
[5] White House Briefing Room, “Fact Sheet: Biden Administration Jumpstarts Offshore Wind Energy Projects to Create Jobs,” March 29, 2021.
[6] American Clean Power Association. U.S. Offshore Wind Industry Status Update 2021. 2021.
[7] One megawatt hour is equivalent to 1,000 kilowatt hours. Note that $0.10/kWh is equivalent to 10¢/kWh or $100/MWh.
[8] Beiter, P., W. Musial, A. Smith, L. Kilcher, R. Damiani, M. Maness, S. Sirnivas, T. Stehly, V. Gevorgian, M. Mooney, G. Scott. 2016. A Spatial-Economic Cost Reduction Pathway Analysis for U.S. Offshore Wind Energy Development from 2015–2030 (Technical Report), NREL/TP-6A20-66579. P. viii.
[9] Beiter, P., W. Musial, A. Smith, L. Kilcher, R. Damiani, M. Maness, S. Sirnivas, T. Stehly, V. Gevorgian, M. Mooney, G. Scott. 2016. A Spatial-Economic Cost Reduction Pathway Analysis for U.S. Offshore Wind Energy Development from 2015–2030 (Technical Report), NREL/TP-6A20-66579. P. xv.
[10] Beiter, P., W. Musial, A. Smith, L. Kilcher, R. Damiani, M. Maness, S. Sirnivas, T. Stehly, V. Gevorgian, M. Mooney, G. Scott. 2016. A Spatial-Economic Cost Reduction Pathway Analysis for U.S. Offshore Wind Energy Development from 2015–2030 (Technical Report), NREL/TP-6A20-66579. P. viii.
[11] For more detailed information about capacity factor along the east coast, see the Department of Energy 2016 National Offshore Wind Strategy Report. p. 8; For a detailed map of offshore wind speed at 100 meters, see US Department of Energy, Office of Energy Efficiency & Renewable Energy. United States - Land Based and Offshore Annual Average Wind Speed at 100 Meters.
[12] Dominion Energy. “Dominion Energy Completes Construction of First Offshore Wind Project in U.S. Federal Waters.” Dominion Energy Media Room, 29 June 2020.
[13] Sweeney, Darren. “Dominion Energy Announces 3-Phase, 2,640-MW Offshore Wind Project.” S&P Global Market Intelligence, 19 Sept. 2019.
[14] Ørsted. Orsted to Pioneer Deployment of GEs next Generation Offshore Wind Turbine. 19 Sept. 2019.
[15] Capacity factor estimates the actual energy generation by the project, as opposed to the amount of energy it could generate if it were running continuously at maximum capacity. For example, a 12 MW turbine can generate a maximum of 12 MW but will always not generate 12 MW.
[16] General Electric. “World’s Most Powerful Offshore Wind Turbine: Haliade-X 12 MW | GE Renewable Energy.” Gepower-Renewables-V2, webpage. Accessed 2 Apr. 2020.
[17] Gheorghiu, Iulia. “Orsted Taps GE for 50% More Efficient Turbines in New Jersey, Maryland Offshore Wind Projects.” Utility Dive, 23 Sept. 2019.
[18] U.S. Department of Energy. Wind Vision: A New Era for Wind Power in the United States. Chapter 3, Impacts of the Wind Vision.
[19] With many projects staggering construction start dates across multiple tranches, it is likely that the total project would last closer to 40 years, from breaking ground on the first turbine in the first tranche to decommissioning the final turbine decades later.
[20] Steffen, Bjarne. “Estimating the Cost of Capital for Renewable Energy Projects.” Energy Economics, vol. 88, May 2020, p. 104783. doi:10.1016/j.eneco.2020.104783.
[21] National Renewable Energy Laboratory (NREL). 2019 Annual Technology Baseline: Electricity. Project Finance Impact on LCOE. 2019; Stehly, Tyler, et al. 2017 Cost of Wind Energy Review. NREL/TP-6A20-72167, Sept. 2018.
[22] Consolidated Appropriations Act, 2021. H.R. 133, 116th Cong. (2020).
[23] U.S. Department of Energy, Office of Energy Efficiency & Renewable Energy. WINDExchange: Production Tax Credit and Investment Tax Credit for Wind. Webpage. Accessed 29 Dec. 2020.
[24] Internal Revenue Service. Notice 2021-05, “Beginning of Construction for Sections 45 and 48; Extension of Continuity Safe Harbor for Offshore Projects and Federal Land Projects.”
[25] Davis, Jefferey, et al., “Solar and Wind Tax Credits Extended, Again.” Energy Forward, 7 January, 2021.
[26] Navigant Consulting, Prepared for U.S. Department of Energy. Offshore Wind Market and Economic Analysis. 2014.
[27] Buatois, Aymeric, et al. “Analysis of North Sea Offshore Wind Power Variability.” Resources, vol. 3, May 2014, pp. 454–70, doi: 10.3390.
Segal, Katie and Henry Lee. “Offshore Wind in the Eastern United States.” December 2021