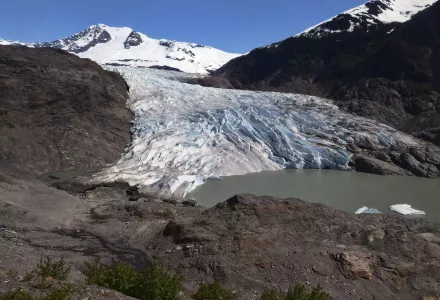
Editor's Note: This brief was originally prepared for and presented at the Harvard/DHS Workshop on Impacts and Policy Challenges from Rapid Climate Change in Alaska, co-hosted by Harvard Kennedy School’s Arctic Initiative and the Science and Technology Directorate of the U.S. Department of Homeland Security on May 8, 2023, in Cambridge, MA. It has been edited from its original form.
The Earth’s surface north of the Arctic Circle, which includes nearly a third of Alaska, is warming 3-4 times faster than the global average.1 Alaska as a whole is warming twice as fast as the lower 48 states.2 As is true for most of the manifestations of anthropogenic climate change, moreover, the extremes of temperature are growing faster than the averages: the highest-ever temperature north of the Arctic Circle—100.4°F—was recorded in Verkhoyansk, Siberia, in June 2020;3 Anchorage reached an all-time high of 90°F on July 4, 2019;4 and Utqiavgik, Alaska, the northernmost U.S. city, reached an all-time winter high of 40°F in December 2022.5
The reasons warming is so fast in the Far North are quite well understood scientifically. The most important factor is the ice-snow-albedo feedback, in which warming reduces the area of land and water covered by ice and snow, which means less reflectivity and more absorption of incident solar energy at the surface, hence further warming.
Sea Ice
The biggest contributor to the ice-snow-albedo feedback is the shrinkage of the area covered by sea ice. That coverage has fallen by about 50%, at its annual minimum in September, since accurate satellite monitoring of the ice began in 1979; sea-ice thickness has decreased even faster—by about three quarters since 1979.6 That portends faster shrinkage in ice-covered area going forward, because thin ice disappears faster than thick under warming. The Arctic Ocean could be completely ice-free in summer as early as 2040.7
Arctic sea-ice decline has multiple important consequences within the region besides the acceleration of warming:
- The summer increase in open water and year-round prevalence of thinner ice mean increased possibilities for marine shipping across the Far North as well as for open-water fisheries and exploitation of seabed resources. Satellite observations confirm that shipping has, in fact, been increasing in both the national economic zones and the Arctic high seas.8 Expanded marine traffic brings economic benefits but also more Arctic pollution by ship emissions and increased demands for traffic monitoring, search and rescue, environmental cleanup, and shore-based infrastructure to support it all.
- Storms whip up waves on waters previously covered by ice, and the absence of ice at the ocean’s edge lets the waves and storm surge impact previously protected shorelines. The result, abetted by local sea-level rise in areas where coastal uplift has not cancelled it out, is accelerated coastal erosion and infrastructure damage.
- The retreat of sea ice is a contributing factor to ocean acidification in the far North, which is proceeding three times faster than acidification in more temperate waters.9 This is impacting marine organisms that make their shells or skeletons from calcium carbonate—including clams, mussels, crabs, and some zooplankton—with potential consequences for subsistence food gathering and commercial crustacean harvests.
- Sea ice supports feeding, birthing, and hunting by seals, walruses, and polar bears, as well as nourishing marine food webs built on algae on the underside of the ice.10 On the other hand, increasing penetration of sunlight into the water column where sea ice has disappeared tends to increase total biological productivity. As a result, even though the subsistence hunting on which coastal Indigenous populations depend is being adversely affected by sea-ice retreat, the potential for some Arctic Ocean fish harvests might be enhanced by the reduction in sea ice if the tendency to increase open-water productivity outweighs the harm from acidification.
The decline of Arctic sea ice also has significant consequences outside the region. A major driver of Northern Hemisphere weather patterns and ocean currents is the temperature difference between the Arctic and the equator—a difference that is shrinking because the Arctic is warming faster. With sea-ice decline interacting in complex (and still not wholly understood) ways with other changing influences on weather patterns—the incidence of strong El Niños, for example—the result has been changes in the circumpolar jet stream and atmospheric blocking patterns that are increasing episodes of extreme temperature and precipitation across much of North America and Eurasia.11
Land Ice and Sea-Level Rise
Arctic and near-Arctic coastal and mountain glaciers are losing ice under the rapid warming in the region, as is the Greenland Ice Sheet (GIS). Unlike sea ice, however, which is floating and therefore does not add to sea level when it melts, land ice lost from Arctic glaciers and from the GIS is a major contributor to global sea-level rise. (Other contributors include discharge from the Antarctic Ice Sheet and glaciers in the midlatitudes and tropics, “mining” of terrestrial groundwater stocks, and thermal expansion.) The decline of land ice can also affect local weather patterns, river flow and turbidity, and access to terrestrial resource deposits.
The GIS has lost more than 5000 gigatons of ice since the year 2000, and Alaska’s glaciers lost about 1500 gigatons in the same period.12 The rates of loss from both are increasing. Recent studies have shown why: the combination of surface melt, lubrication of flow toward the sea by melt water that has penetrated to the bottom of the ice, and erosion on the underside of coastal glaciers where they meet the ocean is a potent accelerator of ice loss.
Global mean sea level (GMSL) has increased about 18 centimeters over the past hundred years: the rate of increase today, however, is more than twice what it was in the 1990s and six times faster than it was 100 years ago.13 Further increase in the rate is expected as the ice losses from both Greenland and Antarctica continue to grow—perhaps dramatically. Mid-range projections anticipate another 0.5-meter rise in GMSL by 2050, reaching one meter by 2100; high-end numbers are two times larger.14
Sea-level rise is not uniform geographically, however. The ocean is not a bathtub but sits on a nonuniformly heated, rotating sphere on which equalization of water levels is further inhibited by gravitational anomalies and the interactions of ocean currents and tides with landforms. Local sea level can also be affected by uplifting or subsidence at continental and island margins. In the case of Alaska, local sea-level rise has been and is projected to be significantly less than the GMSL increase along most of the southern coast of the state and along the Aleutians, about the same as GMSL or slightly less along the state’s west coast, and moderately larger than GMSL along the northern coast.15
The consequences of changes in local sea level across the Arctic are many: acceleration of the coastal erosion associated with sea-ice retreat and permafrost thaw (with all the associated complications for coastal settlements, infrastructure, and military bases); impacts on estuaries and coastal wetlands; saltwater intrusion into freshwater aquifers; and more.
Holdren, John. “The Science of Rapid Climate Change in Alaska and the Arctic: Sea Ice, Land Ice, and Sea Level.” Belfer Center for Science and International Affairs, Harvard Kennedy School, August 16, 2023
- Rantanen, Mika et al. 2022. “The Arctic Has Warmed Nearly Four Times Faster than the Globe since 1979." Communications Earth and Environment 3, no. 168: 1-8. https://doi.org/10.1038/s43247-022-00498-3.
- Thoman, Rick and John Walsh. 2019. Alaska’s Changing Environment. International Arctic Research Center, University of Alaska Fairbanks.
- Erdman, Jonathan. 2022. “It Just Hit 100 Degrees Fahrenheit in Siberia, the Hottest Temperature on Record So Far North in the Arctic.” Weather.com. June 21. https://weather.com/news/climate/
news/2020-06-21-siberia-russia-100-degrees-heat-record-arctic - Di Liberto, Tom. 2019. “High temperatures smash all-time records in Alaska in early July 2019.” Climate.gov. July 16. https://
www.climate.gov/news-features/event-tracker/high-temperatures-smash-all-time-records-alaska-early-july-2019 - Livingston, Ian and Jacob Feuerstein. 2022. “Alaska’s northernmost city posts warmest winter temperature on record.” The Washington Post. December 6.
- Voosen, Paul. 2020. “New feedbacks speed up the demise of Arctic sea ice.” Science, vol. 369, No. 6507, pp 1043-44. 28 August.
- Guarino, Maria-Vittoria et al. 2023. “Sea-ice-free Arctic during the Last Interglacial supports fast future loss.” Nature Climate Change, vol. 60, pp 928-932 (October). https://doi.org/10.1038/s41558-020-0865-2
- Druckenmiller, M.L., R.L. Thoman, and T.A. Moon, Eds. 2022. Arctic Report Card 2022. https://doi.org/10.25923/yjx6-r184.
- Qi, Di et al. 2022. “Climate change drives rapid decadal acidification in the Arctic Ocean from 1994 to 2020.” Science, vol. 377, pp 1544-50 (30 September). https://www.science.org/doi/10.1126/science.abo0383
- Lannuzel, Delphine et al. 2020. “The future of Arctic sea-ice biogeochemistry and ice-associated ecosystems.” Nature Climate Change, vol. 10, pp 983-992 (November). https://doi.org/10.1038/s41558-020-00940-4
- Cohen, Judah et al. 2021. “Linking Arctic variability and change with extreme winter weather in the United States.” Science vol. 373, pp 1116-1121 (3 September). https://doi.org/10.1126/science.abi9167. Cuomo, D. et al. 2018. “The influence of Arctic amplification on mid-latitude summer circulation.” Nature Communications, 9:2959, 12 pp (20 August) https://doi.org/10.1038/s41467-018-05256-8
- Otosaka, Ines N. et al. 2023. “Mass balance of the Greenland and Antarctic ice sheets from 1992 to 2020.” Earth System Science Data, vol. 15, pp 1597-1616. https://doi.org/10.5194/essd-15-1597-2023. Thoman, R., M. L. Druckenmiller, and T. Moon, Eds., 2022. “State of the Climate in 2021, Chapter 5: The Arctic”. Bull. Amer. Meteor. Soc., 103 (8), S257–S306, https://doi.org/10.1175/BAMS-D-22-0082.1
- World Meteorological Organization. 2023. State of the Global Climate 2022. WMO-No. 1316. https://library.wmo.int/doc_num.php?explnum_id=11593
- Sweet, W.V., et al. 2022. Global and Regional Sea Level Rise Scenarios for the United States. NOAA Technical Report NOS 01. National Oceanic and Atmospheric Administration. 111 pp. https://oceanservice.noaa.gov/hazards/sealevelrise/noaanostechrpt01-global-regional-SLR-scenarios-US.pdf
- Ibid.